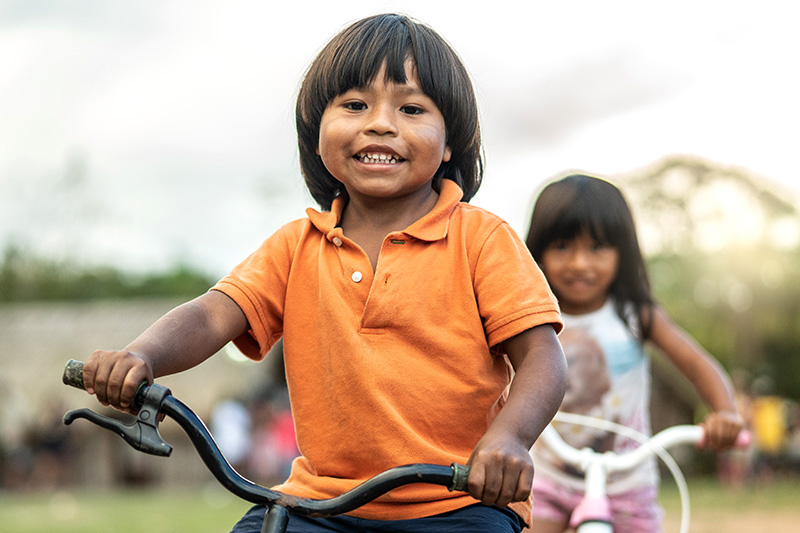
Credit: Stock Image
Eight years ago, NIH announced a collaboration with the Bill & Melinda Gates Foundation (BMGF) to develop solutions for a variety of global health challenges. One priority is the study of brain development in children around the world, particularly those who live in low- and middle-income countries (LMICs) who are exposed to a variety of risk factors such as inadequate nutrition and lack of access to education. Experts from NIH and BMGF participate in joint maternal and child health working groups to tackle these issues.
Extending the Reach of Global Research
“Advancements in science and health should reach pregnant women, children, and families around the globe,” said Vesna Kutlesic, Ph.D., director of NICHD’s Office of Global Health and coordinator of three NIH-BMGF working groups on healthy pregnancy outcomes, growth and nutrition, and neurodevelopment. “We’re part of a global community, and research should aim to improve health and developmental outcomes of children in culturally diverse contexts. In the United States, we have also benefited from research discoveries made in other countries.”
By mapping changes in the brain over time, researchers hope to identify critical developmental windows or processes that may lead to new insights and improved interventions for neurological conditions, disorders, and diseases. As key risk factors are identified, healthcare providers have a better opportunity to intervene early and prevent adverse health outcomes or limit their effects.
However, better assessment approaches are needed to study the brain, especially in communities lacking adequate healthcare services. Some treatment facilities in LMICs simply do not have the infrastructure to support bulky imaging devices that require substantial electricity to power. Consequently, BMGF and NIH researchers are testing portable magnetic resonance imaging (MRI) scanners at study sites around the world. These mobile scanners
fit into the back of a truck and can be transported to difficult-to-reach communities within the United States and abroad.
“The ability to bring a portable scanner directly to individuals represents a paradigm shift in data collection, allowing more diverse and inclusive study populations to be enrolled and followed, as well as expanding access to potential patient populations. Our portable imaging solution addresses this access gap,” said Sean C. L. Deoni, Ph.D., the BMGF senior program officer who is leading research on portable MRI scanners.
Broadening the Use of Neuroscience Tools
Research led by NICHD’s Peter J. Basser, Ph.D., addresses another scientific gap. Dr. Basser, who heads the Section on Quantitative Imaging and Tissue Sciences, is a bioengineer whose invention, diffusion tensor magnetic-resonance imaging (DTI), has helped advance the study of neuroscience and brain development over the last three decades. He is part of the NIH-BMGF neurodevelopment working group and learned that one of his inventions has a role in this global research.
Dr. Basser and his lab developed a diffusion MRI phantom, a device to calibrate MRI scanners to ensure the quality and accuracy of images. This quality control is especially important for neurosurgeons who need to avoid sensitive areas of the brain—for example, areas that control touch, taste, or mobility—during neurosurgery. It’s also useful for oncologists who need to evaluate whether a cancer patient’s therapy is working. NIH holds the patent for this device, and companies have licensed the technology to bring it to the clinic.
During discussions with the NIH-BMGF neurodevelopment working group, Dr. Basser learned that a commercial MRI phantom was being used across study sites to calibrate the portable MRI scanners. The phantom helps ensure that data collected on scanners from different sites are accurate and comparable.
“We initially developed this calibration device to meet a need in the neuroscience community,” said Dr. Basser. “I was pleasantly surprised to see the phantom used in a global health study. It’s come full circle.”
An important attribute of the phantom is that it’s made of polyvinylpyrrolidone, or PVP, a stable and non-toxic substance used as a food additive. This means the device is safe and easy to use, store, and ship.
“Our team, Drs. Ferenc Horkay and Carlo Pierpaoli, tried several polymers before PVP, but they shook in the scanner. You don’t want motion like that; you want to suppress it. There are other substances that can do the job from the MRI perspective, such as alkanes, but they produce volatile flammable vapor. You wouldn’t want to drop it, and you can’t ship it easily,” explained Dr. Basser. “There aren’t many substances that would fit all these criteria so well.”
The calibration device is also part of a lending library run by the National Institute of Standards and Technology (NIST) and NIH’s National Institute of Biomedical Imaging and Bioengineering (NIBIB). Researchers can borrow the phantom for use in their own studies.
“NICHD has identified, developed, and patented PVP solutions as a diffusion reference. It is remarkably stable and a rarity among any tissue mimics,” shared Stephen Russek, Ph.D., Project Leader for Imaging Physics at NIST. “As we work to characterize more medical imaging reference standards, we increasingly appreciate PVP solutions. The NICHD team, through the development of these diffusion standards, has done a great community service that ensures more accurate diagnostics from low-field MRI scanners in LMICs, clinical MRI scanners in U.S. hospitals, and powerful research MRI scanners used for brain research.”
Looking Forward
Dr. Basser, whose lab is developing new imaging and analytic methods to improve data collection and analyses for the NIH and BMGF pediatric neuroscience study, is eager to see this global project progress.
“In the MRI field, we constantly deal with echoes, for instance, ‘echo trains’ in our measurements. This concept applies to my own research—every 5 to 10 years, we see new uses emerge from our technologies. It’s interesting how it all comes back. What will we see in the next echo five years from now?”