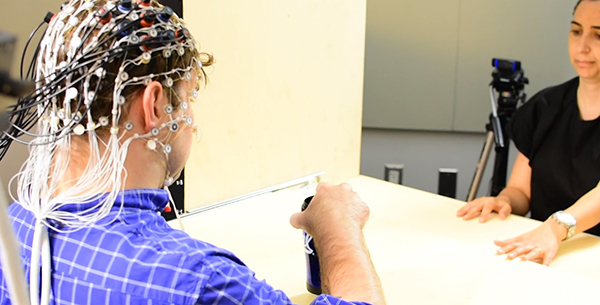
Credit: Jeremy Swan, NICHD/NIH
The brain is one of the largest organs in the body, but studying the brain is not as easy as you might think. There are limitations to what researchers can do. Standard imaging technology, such as MRI, require that a person lie still in a scanner for long periods of time, something that can be challenging for children and those with disabilities. However, newer portable imaging systems may help researchers overcome these challenges to conduct studies more easily.
NICHD’s Amir Gandjbakhche, Ph.D., leads a team that is pioneering the use of portable imaging technology to study human development and health conditions, including brain injuries and disorders. His laboratory, the Section on Analytical and Functional Biophotonics, is one of only a handful at NIH that focuses on biophotonics—using the energy and properties of light to study what happens in organs, tissues, and cells.
“We use light in a non-invasive way to measure activity in tissues like the brain and placenta,” said Dr. Gandjbakhche. “If you hold a flashlight to your finger, you’ll see that the light will pass through a short distance and glow red because of the hemoglobin in your blood. We can obtain measurements from these readings and correlate it with tissue activity.”
Dr. Gandjbakhche’s lab specializes in a type of non-invasive imaging called functional near-infrared spectroscopy (fNIRS). The method uses near-infrared light to measure brain activity by detecting changes in blood flow. These changes are color-coded to show regions with higher (red) or lower (blue) brain activity. While the technology has been around for more than two decades, the lab is developing new ways to analyze fNIRS data and combine them with other types of measurements.
Brain activity measured in two different scenarios using fNIRS. The different colors show where brain activity is high (red) or low (blue), based on the level of oxyhemoglobin measured in blood. Credit: https://www.ncbi.nlm.nih.gov/pmc/articles/PMC6236239/
Currently, the lab is piloting their methods in adult volunteers. It aims to start a study in children by summer 2019. They’re recruiting families with infants who are 9 to 12 months old for a clinical study, Mirror Neuron Network Dysfunction as an Early Biomarker of Neurodevelopmental Disorder. The “mirror network” is a unique set of brain cells that are active when a person completes a task and when a person watches someone else completing the same task.
For the pilot study and clinical trial, the lab is developing a “fusion analysis” that combines fNIRS with another type of non-invasive brain measurement called electroencephalogram (EEG), which records the brain’s electrical activity. Combining these two techniques allows researchers to study details of brain activation that may not be possible using one type of measurement alone. For example, fNIRS offers better spatial resolution, meaning it’s easier to identify regions of activation. EEG offers better temporal resolution, giving a better idea on the timing of activation.
Helga De Oliveria Miguel, Ph.D., and Doug Harrison demonstrate how they use fNIRS in their work.
Credit: Jeremy Swan, NICHD/NIH
The portability of the imaging devices allows Dr. Gandjbakhche and colleagues to study brain function while subjects perform tasks in realistic settings. This portability also enables the researchers to study the mirror neuron network. Researchers believe this network plays a vital role in social interactions, but it is difficult to study—especially in children—because of the requirements of traditional imaging techniques.
The fusion analysis will help identify potential differences in brain activity patterns between typically developing toddlers and those at risk for developmental delays, like those with autism spectrum disorder. Such differences could serve as markers to assist with screening and diagnosis. Early diagnosis allows parents and healthcare providers to offer support and interventions for children as early as possible, maximizing each child’s potential and success.
The lab is also using fNIRS to study a set of rare diseases called urea cycle disorders , which affect brain development and executive function. Executive function refers to brain processes that help a person concentrate, plan, reason, and problem solve.
Dr. Gandjbakhche notes that these projects are only possible because of an interdisciplinary team of scientists and students who work closely together. Colleagues Helga De Oliveira Miguel, Ph.D., and Emma Condy, Ph.D., have psychology backgrounds and provide behavioral expertise. Hadis Dashtestani, M.S., is a computer scientist who analyzes the data. Kosar Khaksari, Ph.D., and Afrouz Anderson, Ph.D., refine the devices and analyses using their biomedical engineering expertise. Aisling Casey, Joy Cui, Doug Harrison, and John Millerhagen are trainees who help with data collection and research setup.